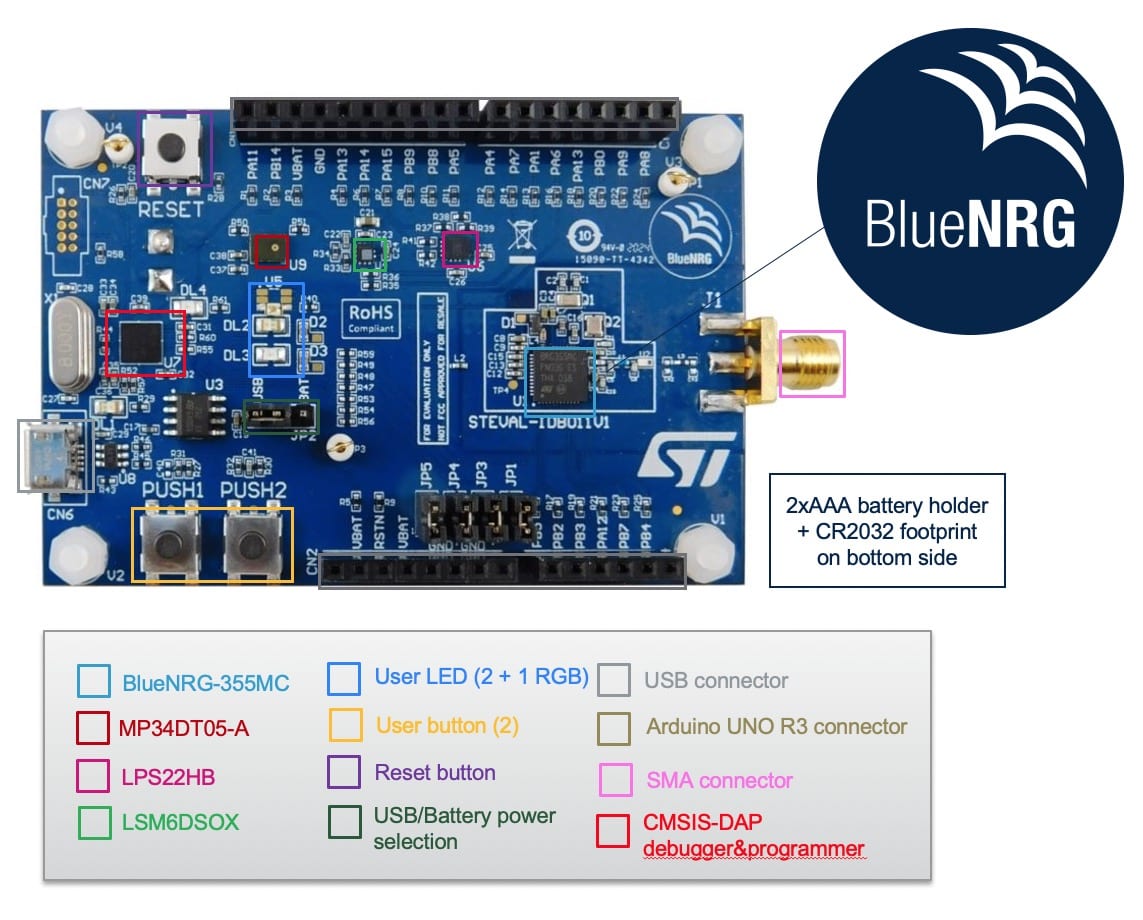
The process engineering teams go through to select a Bluetooth SoC is often complex. Many factors may influence a decision, from costs to past experiences, performance, and ease-of-use. Hence, to help teams evaluate the BlueNRG-LP faster, we also released updates to our software and development tools. For example, developers can use the new STEVAL-IDB011V1 BlueNRG-LP evaluation board with the BlueNRG Navigator GUI. The software can upload example applications, thus helping managers see what to expect from our new SoC.
The number of demo projects available from BlueNRG Navigator GUI is quite large. Out of the 20 or so applications, developers can rapidly test the SoC’s ability to connect to up to 128 devices. They can also try advertising extensions by broadcasting to eight channels instead of the traditional three. Similarly, a project showcases the higher throughput available while another offers longer range capabilities. Hence, engineers have numerous application starters that can drastically hasten the development of their proof-of-concept.
ST also released a new version of the BlueNRG current consumption tool. The utility offers a convincing graphical representation of the low-power capabilities of our new device to sway decision-makers. Developers may also decide to test the new device by porting code running on previous BlueNRG SoCs. Since it’s merely a matter of moving from a Cortex-M0 to a Cortex-M0+, the process is relatively straightforward.
On the opposite end of the LE 2M PHY, Bluetooth 5.0 offers greater coverage. Unfortunately, many engineers often overlook this long-range functionality because they underestimate its potential. BlueNRG-LP offers LE Coded PHY, enabling far greater distances between two Bluetooth devices without requiring an additional power amplifier. ST’s real-world tests, which used existing development boards and applications, reached 1.3 kilometers (0.8 miles).
The increase in range is possible because LE Coded PHY uses, among other things, Forward Error Correction, which adds additional bits to each packet. However, data redundancy leads to a lower bandwidth of 125 kbps. Another feature that helps avoid interferences is Channel Selection Algorithm #2 (CSA #2). While CSA #1 could only hop between 37 channels, CSA #2 has 65,535 channels at its disposal. This large selection helps avoid collisions and fading effects. Whether there are a lot of devices nearby, or great distances between them, CSA #2 increase the network’s reliability. Ultimately, the longer-range is possible because Bluetooth 5.0 better handles the background noises that inevitably plague a signal when covering great distances.
Link Budget
Engineers working on Bluetooth applications always run a link budget analysis, a design aid that helps them anticipate overall performance. Put simply, it ensures designers can foresee specific issues, such as an insufficient signal strength incapable of reaching the receiver. In most textbooks, a link budget analysis uses the following equation:
Received power (dBm) = transmitted power (dBm) + gains (dB) − losses (dB).
However, engineers work today with data sheets that almost always give the transmit power (TX) and receiver’s sensitivity level (RX). Hence, most real-world projects define their link budget according to the following equation:
| Link Budget (dB) | = TX Power (dBm) – Sensitivity level (dBm).
The BlueNRG-LP has a TX power capable of reaching +8 dBm (programmable in 1 dBm steps) and an RX sensitivity of -104 dBm at 125 kbps or -97 dBm at 1 Mbps. Hence with a link budget of 112 dB and 105 dB, the new ST SoC has the largest link budget in the industry. As a result, engineers can anticipate better performances at identical power consumption, compared to devices with a lower link budget.
Thwarting Hackers
Security is another crucial aspect that engineers focus on when designing a system. Consumers are a lot more sensitive to issues of privacy and protection against threats. As a result, teams looking to build a Bluetooth system look at features that shield users and data. BlueNRG-LP provides answers to these critical engineering challenges. One of them is the presence of a secure boot loader that checks the firmware’s signature before launching it. Such measure protects against rootkits or low-level attacks. Developers can also disable SWD and UARTaccess to protect the Flash. Similarly, 1 KB of memory is one-time-programmable, to guarantee its integrity. Hence, in theory, a hacker with access to the device wouldn’t be able to clone or modify its content.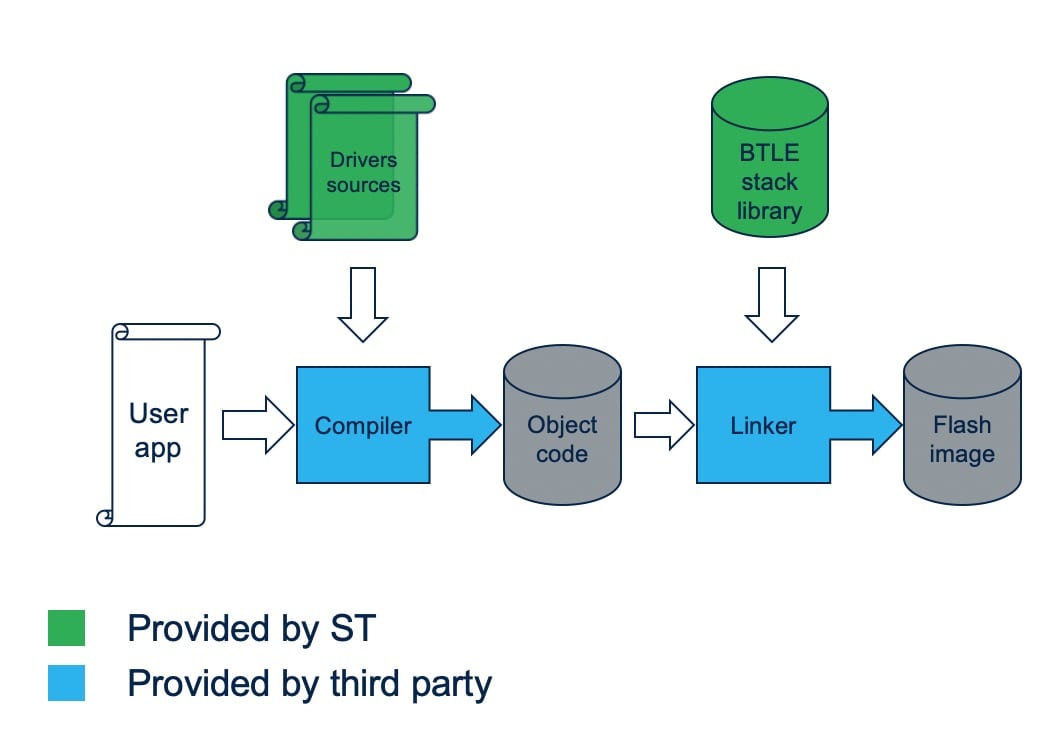
More Computational Throughput and a Lower Power Consumption
Developers must find ways to improve performance, accuracy, and user experience. To solve this challenge, engineers often turn to a device with more computational throughput. However, many Bluetooth end products must have a low power consumption to preserve battery life. Engineers ought, therefore, to find a way to reconcile these seemingly contradictory requirements.
The BlueNRG-LP offers a new solution to this challenge. Thanks to its higher frequency and more powerful architecture, the SoC now supports more complex algorithms. These processes, built on top of MEMS and voice libraries, are highly popular in embedded systems. Moreover, ST also provides a free-of-charge Bluetooth-SIG certified Mesh stack. As a result, it becomes very easy to cover large areas and reach up to 126 hops or 32,000 nodes.
Yet, despite a higher frequency and more memory, the BlueNRG-LP features a lower power consumption than its predecessors. Its peak current in transmission is 4.3 mA (0 dBm) while the BlueNRG-2 needs 8.3 mA (-2 dBm). Similarly, the new device peaks at 3.4 mA in RX (at sensitivity level), whereas the previous generation demands 7.7 mA. The improvements in power consumption are also significant when idle. The BlueNRG-LP needs only 0.6 µA in DEEPSTOP mode with full RAM retention. On the other hand, the BlueNRG-2 requires 9.5 µA, despite having less RAM. All these improvements serve as a testimony to our latest silicon optimizations and improved implementations.